I will always remember the moment which first sparked my interest in neuroscience. It was a rainy day in Oxford – it poured as we stepped off the bus. We had arrived at the University Department for Neuroscience. After being introduced to a group of researchers we were given an extensive tour of the facilities. As an A-level student, the visit was my first encounter with a fully functioning research lab. At its close, the visit left a resounding impression on many of us, and personally I remember becoming immediately interested in the prospect of studying neuroscience at university.
The lab was at least 15 strong, yet they continually reminded us that they were investigating a tiny piece of a puzzle which has been studied by generations of brilliant minds. The interconnection issue; how does the structure of our brain, from single neurones to complex circuits, relate to function. It was their enthusiasm for such a complex question that sparked my own interest. Whether you’re a member of the general public or an active researcher, it’s easy to forget just how amazing the brain is, either because you’re unaware of the dizzying numbers or, like me, you’ve become transfixed on understanding a small part of brain infrastructure from a very specific angle. I write this post to briefly introduce the structure of the brain to those unfamiliar with it, and to serve as a source of motivation for fellow neuroscientists who spend huge amounts of times with their heads buried in the vast sands of the field.
Let’s start with the building blocks. The human brain has about 100 billion individual neurones with an estimated 200 trillion contacts between them. Remarkably this staggering number of neurones are arranged in such a way that we can effortlessly transition from a walk to a run, respond to sensory stimuli, perceive emotions and learn complex skills such as playing an instrument.
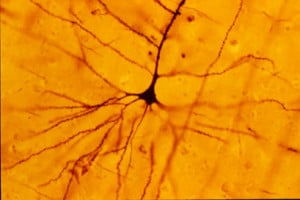
To complicate things further, each neurone is a complex device in its own right; perhaps the most intricate cell type nature has created. Neurones are tree like cells with branching appendages that maximise the receptive surface area for connections from other neurones. To increase the cells receptive area these branched appendages, called dendrites, are covered by many spines. Yes that’s right, branches on branches. The spines accommodate between thousands and tens of thousands of postsynaptic receptors which listen for signals from other neurones.
Dendrites are the targets of thin and long processes from other neurones, called axon collaterals, which typically emerge from the cell body and take a long, convoluted journey to reach a dozen or tens of thousands of nearby and distant neurones. Terminating in close proximity to the cell body and dendrites of other neurones, axons release chemicals that modulate the postsynaptic receptors, evoking a response. This is the basis of neurone to
neurone communication.
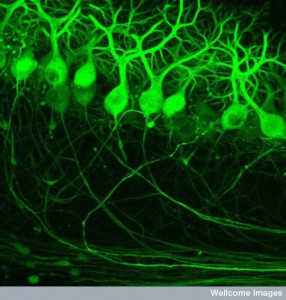
Now we have met the basic structural characteristics that permit neurones to communicate with one another, let’s consider how these change between neurones. We know from detailed imaging studies that the shape and dimensions of a neurone are tailored to fit its role in the brain’s circuitry. Furthermore, different neuronal types are more strongly localised to specific areas of the brain. For example, the cerebellar Purkinje cell epitomises the link between structure and the broader function. Named after their discoverer, Czech anatomist Johann Evangelist Purkinje, these cells are amongst the largest in the brain. Their elaborate tree of dendrites makes them ideally suited to receive input from many other neurones. This is an important feature for a cell which needs a lot of incoming information to effectively coordinate the fine movement of our limbs.
Finally, I want to put aside the physical components and touch briefly on how information is encoded in the brain. Neurones produce single electro-chemical spikes, called action potentials. These electrical discharges result from rapid and well-timed ion movements across the neuronal membrane. Action potentials typically last 2-5ms however they can stretch or compress depending on the amalgamation of ion channels that are incorporated into the membrane. Neurones can repeatedly fire action potentials; the firing frequency depends on postsynaptic inputs and cascades of processes occurring within the cell. Any given neurone may fire just one action potential per second in its resting state. However, when receiving a stimulus from another can increase this firing rate . Neurones can also produce elaborate bursting patterns of action potentials, or can be completely silent.
The brain is complex at every level of its architecture. The billions of neurones, trillions of synapses, an unimaginable number of action potentials and many flavours of ion channel all add layers to its computational capacity. Perhaps even more staggering is that all these components occupy less than a 1 litre volume inside the skull, and are somehow wired together as circuits to convert tiny fluxes of ions to organism-wide behaviours. Now a PhD student, deeply entrenched in a specific research question, I try not to lose sight of the reason I chose to study neuroscience. For me it all comes back to that rainy day in Oxford.
Post by: Adam Watson
References:
‘Ion Channels of Excitable Membranes’ Third Edition- Bertil Hille.
‘Rhythms of the brain’- Gyӧrgy Buzsáki